Many animals, including mammalians, produce a large number of sperm over a long period for reliable reproduction. On the other hand, each sperm correctly replicates and transmits the genetic information to the next generation. The ultimate goal of the Division of Germ Cell Biology is to reveal how living organisms can (or cannot) compromise productivity and fidelity at high levels, which seem to be contradictory by their nature. To this end, we pay special attention to the sperm stem cells, as well as other aspects of spermatogenesis.
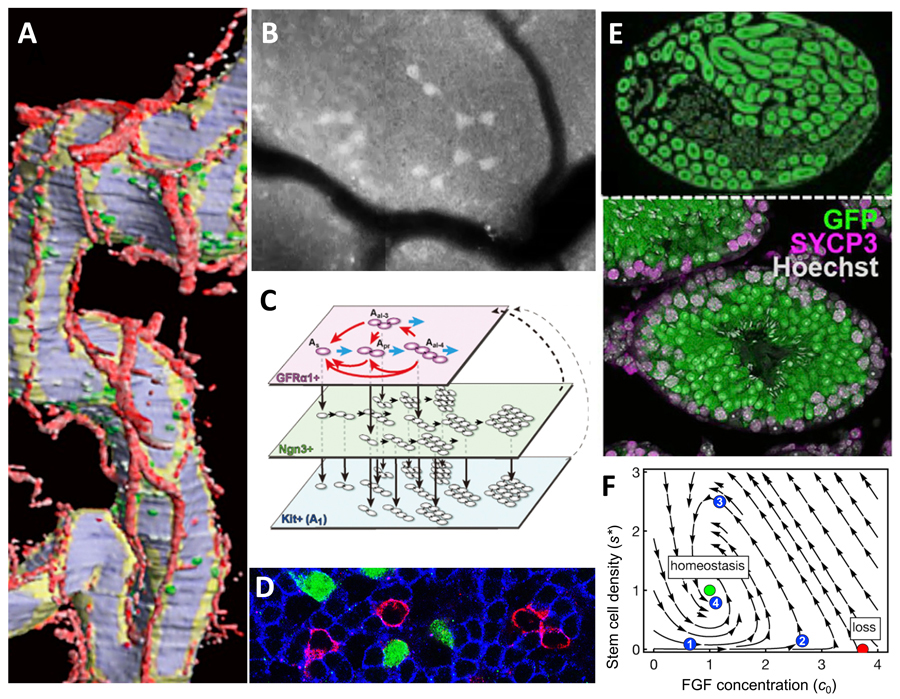
Figure 1. Different looks of mouse sperm stem cells (SSCs)
A. 3D-reconstructed seminiferous tubules, showing SSCs in green, blood vessels in red.
B. An intravital live-imaging filming of GFP+ SSC syncytia undergoing differentiation.
C. Scheme of SSC dynamics in multi-compartment, interconverting singly-isolated and syncytial states.
D. Whole-mount immunofluorescence, showing SSCs (magenta) intermingled with primed (green) and committed (blue) progenies.
E. Sectioned host testis showing repopulated by transplanted donor SSCs (green), augmented by transient RA inhibition.
F. A phase portrait showing the dynamics of homeostatic SSC density regulation in the mitogen competition model.
Research Projects
-
• Mouse sperm stem cell dynamics and regulation
-
• Temperature dependence of mouse spermatogenesis
-
• Mouse germline clonal lineage analysis
-
• Homeostatic regulation of mouse spermatogenesis in the testis
What are the sperm stem cells?
The continual sperm production is supported by the "sperm stem cells," termed formally "SSCs" after spermatogonial or spermatogenic stem cells. SSCs maintain a delicate balance between self-renewal and differentiation to sustain life-long spermatogenesis. It has been a central question which fraction of
spermatogonia, the "early stage of cells before meiosis, are SSCs and where and how they behave in the testis.
The backbone of mammalian SSC research was established from the 1950s to the 1970s, relying on morphological analyses of fixed testis specimens. This led to a proposal of the prevailing stem cell model called the "A
s model." This model proposed that singly isolated spermatogonia (A-single or A
s cells) act as SSCs. On the other hand, all syncytia are all committed to differentiation.
Our lab has introduced the
scale of time using updated technologies,
i.e., live-imaging and pulse-labeling studies, which revealed many different aspects of dynamical behaviors of SSCs (Figure. 1).
As cells and syncytia together comprise a single SSC pool
Our live-imaging studies combined with mathematical and statistical analysis evidenced that SSCs reversibly change their morphology between singly-isolated and syncytia. This is mediated by incomplete cell division and breakdown of syncytia (Nakagawa
et al., Science 2010; Hara et al., Cell Stem Cell 2014).
Cells inclined for differentiation can revert to SSCs
It is generally thought that once stem cells have been destined for differentiation, they never replicate themselves again. We found that such cells maintain the potential to self-renew up to a particular stage of differentiation and revert to SSCs with high frequency, particularly during regeneration after tissue damage (Nakagawa
et al., Developmental Cell 2007).
SSCs follow variable fate behaviors
Stem cells are often thought to invariably undergo asymmetric division, which produces one stem cell and one differentiating cell to maintain homeostasis. However, we found that individual SSCs follow highly variable fates. Still, collectively, they achieve a perfect balance between self-renewal and differentiation at a population level (Klein
et al., Cell Stem Cell 2010; Hara
et al., Cell Stem Cell 2014).
SSCs show active motion in an "open niche"
In many tissues, stem cells receive self-renewing signals in defined niche regions. We found that the mouse testis has no such areas, with SSCs scattered among differentiated cells and moving around actively. Further, their distribution is biased near blood vessels. Such microenvironment is called an "open (or facultative) niche" in contrast to the afore-mentioned "closed (or defined) niche" (Yoshida
et al., Science 2007; Hara
et al., Cell Stem Cell 2014).
SSC heterogeneity underpins the asymmetric fates of SSCs
In an open niche, some SSCs remain undifferentiated while others differentiate in response to broadly distributed signaling molecules. We found that some SSC fractions resist differentiation-promoting Wnt signal by expressing a Wnt-inhibitor, Shias 6. Another subset responds to retinoic acid (RA) by upregulating an RA receptor to differentiate (Ikami et al., Development 2015, Tokue
et al., Stem Cell Reports 2017).
SSCs compete for self-renewal factors in an open niche
We also question the mechanism that maintains a constant SSC density in the open niche of the testis. Evidence suggests that SSC density homeostasis is achieved through competing with each other for limited amounts of self-renewal factors (
e.g., FGFs). This mechanism was proposed as the "mitogen competition model" (Kitadate
et al., Cell Stem Cell 2019).
Periodic differentiation of SSCs
Strikingly, differentiation of SSCs and subsequent differentiation to mature into sperm occur synchronously every 8.6 days. This is called the seminiferous epithelial cycle. We propose that the periodic differentiation is triggered by periodic synthesis and degradation of RA (Sugimoto
et al., Mechanisms of Development 2012).
Clonal analysis and tuning of post-transplantation SSC fate
When SSCs are transplanted into host animals' seminiferous tubules whose germ cells have been depleted, SSCs engraft and regenerate spermatogenesis (Brinster
et al., 1994). What happens during repopulation was largely elusive. We found that donor SSCs follow stochastic fate with significant similarity with homeostasis. We further succeeded in tuning the donor SSC fate to increase the repopulation efficiency, which would promise the practical use of this technology (Nakamura
et al., Cell Stem Cell 2021).
Identification of a tiny, most primitive fraction of SSCs
An important advance gained in FY2021 is that we have detailed gene expression heterogeneity of SSCs (precisely, a population called “undifferentiated spermatogonia”) and their dynamical state transitioning in supporting the homeostatic and continual sperm production (Nakagawa
et al., Cell Reports 2021). This is a collaborative study with Nagasawa (Osaka) and Simons (Cambridge) labs. We identified that a gene
Plvap (plasmalemma vesicle associated protein) is expressed in a tiny, most primitive fraction of mouse SSCs (Figure 2A).
Detailing the heterogeneity of mouse SSCs
Plvap shows overlapping expression with some other genes, including Eomes, Pdx1, Shisa6, and T (brachyury). By dimension reduction of single-cell (sc)RNA-seq data (La
et al., 2018), Plvap
+ cells cluster at the undifferentiated end of SSCs, forming a discrete subfraction of cells expressing established undifferentiated markers, GFRα1 and Id4. In contrast, differentiation-related genes (
e.g., Sox3, Ngn3, and RARγ) is expressed in cells near the opposite end (Figure 2B). Based on these, Figure 2C summarizes the heterogeneous expression of key SSC genes.
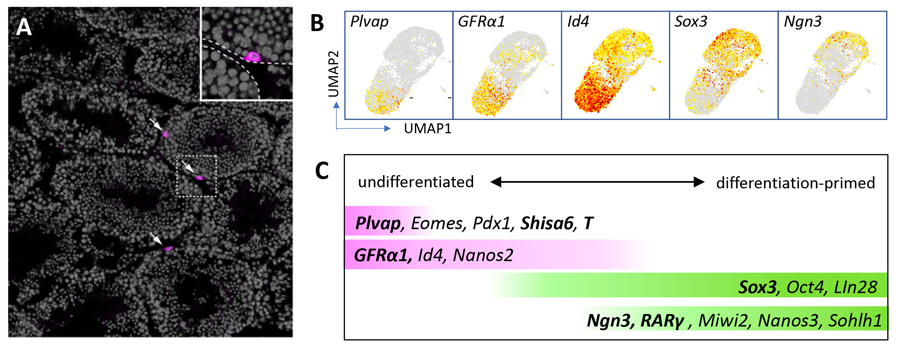
Figure 2. Heterogeneity of SSCs
A. Immunostaining of a mouse testis section for Plvap protein (magenta), with DNA counterstained in gray. Only three cells located on the periphery of seminiferous tubules express Plvap.
B. UMAP analyses of scRNA-seq data showing the expression of indicated genes.
C. A summary of expression profile of a set of genes within SSCs. Genes shown in bald have been analyzed in our lab.
Adopted from Cell Reports 37 (3), 109875.
Contribution of multiple SSC fractions to self-renewing pool in homeostasis
By pulse-labeling using tamoxifen-dependent CreER, Plvap
+, Sox3
+, and Ngn3
+ cells were found to contribute to the self-renewing pool in homeostasis. In particular, after being sparsely labeled with GFP expression, these cells gave rise to cell patches comprised of GFP
+ germ cells in three months (Figure 3).
Interestingly, while the patch-forming efficiency varied between these fractions, the resultant individual patches showed indistinguishable size distribution irrespective of the original cell state. Further, strikingly, the same heterogeneous SSC population was established in every such patch, again, irrespective of the original cell state.
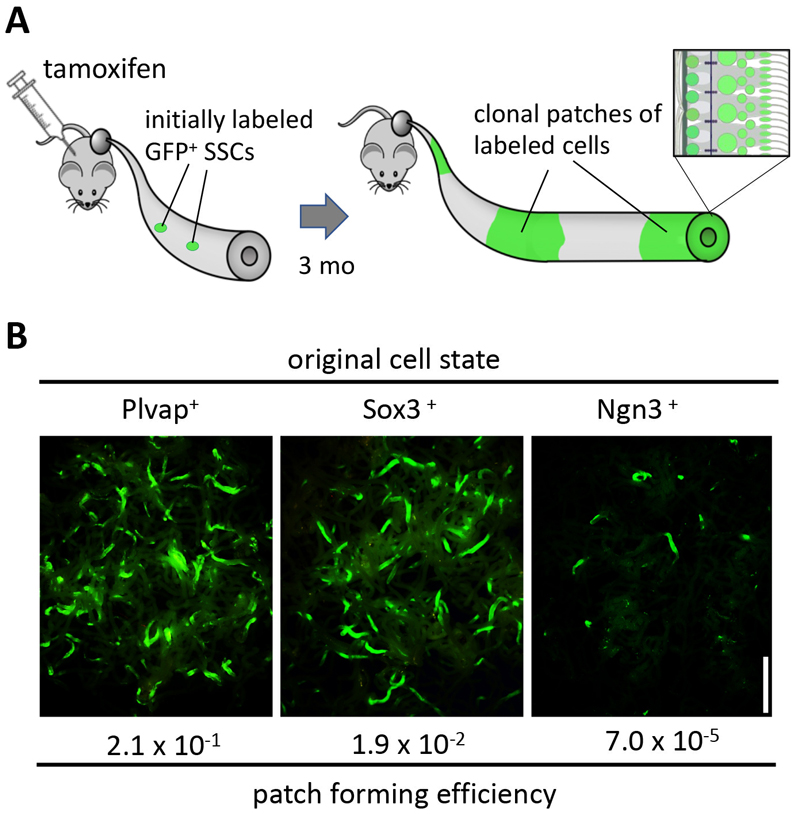
Figure 3. Pulse-labeling of multiple SSC fractions
A. Experimental design addressing the contribution of spermatogonia expressing particular genes to homeostasis, following a pulse-labeling by tamoxifen injection.
B. Results of the pulse-labeling of the Plvap
+, Sox3
+, and Ngn3
+ fractions. Note the differential patch forming efficiencies in contrast to the similarly-looking individual patches.
We reasoned that SSCs transit continually and reversibly between multiple cell states, from the self-renewal-biased Plvap
+ state to the differentiation-primed Ngn3
+ state (Figure 3B). As a result, a heterogeneous SSC pool is always established, which both maintains itself and gives rise to irreversibly committed progenitor cells of Kit
+ spermatogonia as a result of cell division and state transition.
A multistate dynamics: Proposal of an inclusive model of mouse SSCs
Finally, we synthesized a mathematical model that captures the multistate SSC dynamics in homeostasis. This model assumes that the cells undergo cell division and state transition (either differentiation and reversion) in a stochastic (
i.e., probabilistic) manner, following particular rates specific to each state (Figure 4).
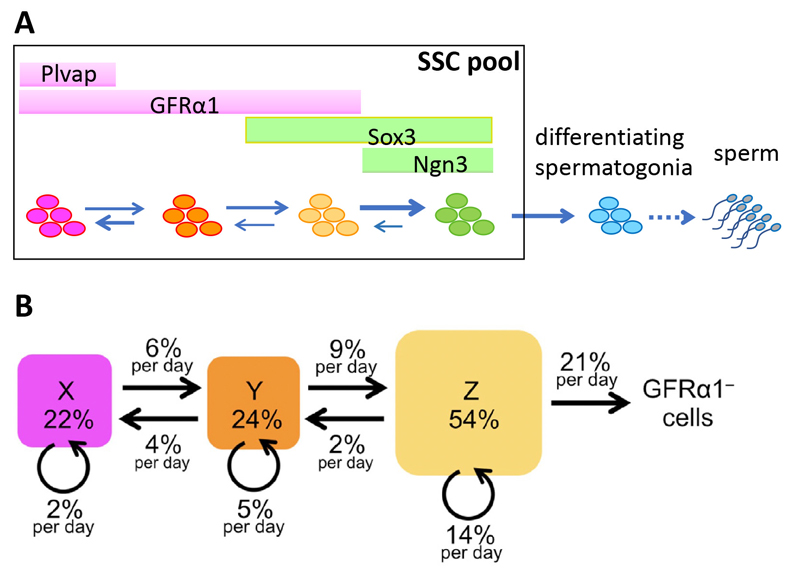
Figure 4. Multistate dynamics of mouse SSCs
A. A general perspective of the multistate dynamics, in which SSCs transit between multiple states reversibly to form a single SSC pool.
B. A numerically fit dynamics of mouse SSCs in homeostasis. The flows of cells between cell states and cell division at respective states are shown in values relative to the total GFRα1
+ cells per day. Note that the GFRα1
– (Ngn3
+) to GFRα1
+/Sox3
+ state is not defined due to its low frequency in this model. Adopted from Cell Reports 37 (3), 109875.
Interestingly, such a multistate dynamics is found capable of saving the cell divisions in the self-renewing pool in producing a certain number of differentiating cells. This is due to the differential cell division frequencies between the states; the more primitive the state is, the less frequently the SSC divide. See Nakagawa
et al., Cell Reports 2021 for detail.
Furthermore, this quantitative model can explain the seemingly contradictory models proposed for the SSC dynamics, and may provide inclusive insights into this long-held problem in the field of SSC research.