The transport of specific mRNAs and local translation control by RNA granules in neuronal dendrites are crucial components of gene expression, enabling precise dendritic protein synthesis at the right time and place within neurons. This system plays a vital role in regulating where stable synaptic connections are formed, thereby supporting long-term neural networks and memory formation. Our lab is dedicated to uncovering the mechanisms and functions of mRNA transport and local translation in neuronal dendrites.
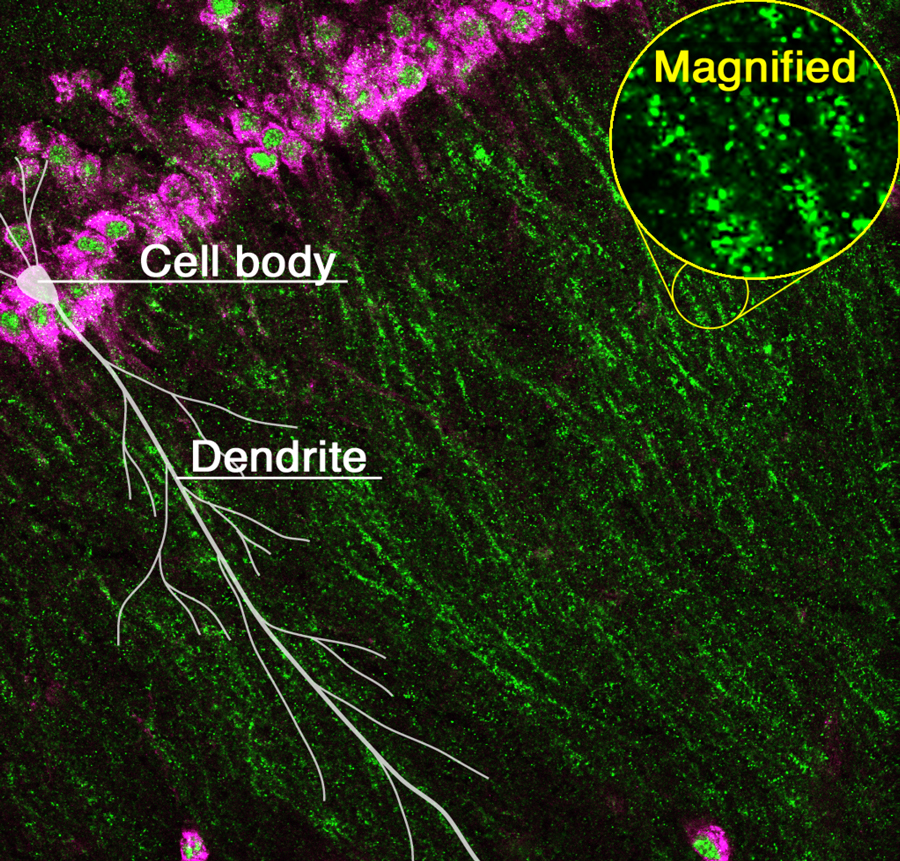
RNA granules (green) transported from the cell body (magenta) to dendrites in the hippocampus of the mouse brain.
Research Projects
-
• Mechanism of local translation and synapse formation regulated by mRNA transport to neuronal dendrites
-
• Comprehensive identification of proteins and mRNAs in neuronal RNA granules using advanced omics techniques
-
• Mechanisms underlying RNA granule dynamics and local translation changes in response to neuronal activity
-
• Role of mRNA m6A modification in regulating local translation in neurons
-
• Contribution of expanded intrinsically disordered regions in vertebrates to the evolution of higher brain functions
Specific mRNAs are recruited into “RNA granules” and transported to dendrites. RNA granules are membraneless organelles formed by liquid-liquid phase separation (LLPS) of RNA-binding proteins and mRNAs, which further recruit other factors such as ribosomes. They mediate the transport of mRNAs to the vicinity of synapses and synaptic stimulation-dependent local translation of the cargo mRNAs (Figure 1). We are currently using mice to research the mechanism of RNA granule assembly, RNA granule factors and their phase behavior regulating mRNA transport and local translation, their target mRNAs, and the roles of the locally synthesized proteins, so we can attain a better understanding of their relationship to the formation of synapses and neural networks, memory, learning, and behavior.
In addition to the physiological function, RNA granules are linked to neurodegenerative diseases such as amyotrophic lateral sclerosis (ALS) and frontotemporal lobar degeneration (FTLD). The basis of these diseases is thought to be the accumulation and aggregation of disease-causing proteins such as FUS and TDP-43 in RNA granules. Thus, our research also aims to reveal the effects of such protein aggregation on mRNA transport and local translation.
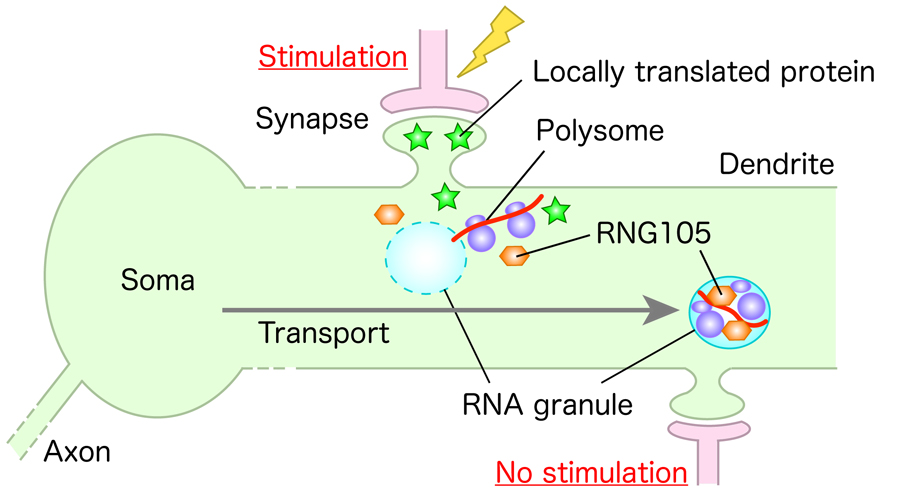
Figure 1. A model for local translation in neuronal dendrites. Specific mRNAs are recruited into RNA granules and transported to dendrites. Translation of the mRNAs is induced locally upon synaptic stimulation, which modifies local postsynapses to regulate synaptic connection and network formation.
I. Prion-like domain of ILF3 regulates gene expression and fear memory under chronic stress
LLPS is generally driven by protein intrinsically disordered regions (IDRs) that do not form three-dimensional structures. One class of IDR is the prion-like domain (PrLD), whose propensity to form aggregates has been extensively studied in the context of neurodegenerative diseases. However, little is known about the physiological relevance of PrLDs in the brain.
We focused on an RNA-binding protein NFAR2, which has PrLD at the C-terminus. This PrLD is spliced out in the alternative splicing isoform NFAR1. NFAR1 and NFAR2 are synthesized from the Ilf3 gene, and both possess biochemical activities that they perform in common such as transcriptional regulation and stress-dependent translational inhibition. However, they differ in that NFAR2 alone can concentrate in the nucleoplasm and cytoplasmic RNA granules through PrLD. To investigate the physiological relevance of PrLD, we generated NFAR2ΔPrLD mice, in which a stop codon was introduced into the NFAR2-specific exon. This is considered an ideal strategy for revealing the physiological role specific to PrLD, as the splicing isoform without PrLD is intrinsically present and maintained even after PrLD deletion.
Deletion of the PrLD lost the nucleoplasmic localization of NFAR1/2 and affected the genome-wide profiles of mRNA expression and translation in the brain. In particular, PrLD deletion significantly altered chronic restraint stress (CRS)-induced changes in mRNA expression and translation in the amygdala, a brain region associated with emotional events such as anxiety and fear.
Consistent with the effects on CRS-induced changes in mRNA expression and translation, PrLD deletion affected tolerance of mice to CRS in amygdala-associated learning and memory: conditioned fear memory in wild-type mice was unaffected by CRS, but it was exacerbated in NFAR2ΔPrLD mice due to CRS. These results indicated that PrLD of NFAR2 is responsible for the regulation of stress-responsive gene expression and confers stress tolerance in fear-associated memory formation, providing insights into PrLD’s involvement in chronic stress adaptation.
II. Novel regulation of RNA granule dynamics by pathogenic accumulation of FUS and TDP-43 in RNA granules
FUS and TDP-43 are predominantly localized in the nucleus when cells are healthy, but in neurodegenerative diseases, they translocate to the cytoplasm and accumulate in RNA granules. FUS and TDP-43 prone to aggregate in RNA granules, which is thought to harden RNA granules, affect the function of RNA granules, and impair synapse formation and function. However, little is known about the effects of FUS and TDP-43 on the dynamics of other components of RNA granules.
We overexpressed disease-related FUS and TDP-43 mutants in primary cultured neurons from the mouse cerebral cortex and analyzed their effects on the dynamics of RNA granule components using fluorescence recovery after photobleaching (FRAP) and cell permeabilization assays (Shiina, J. Biol. Chem., 2019). FUS and TDP-43 did not affect the dynamics of most RNA granule components such as Pum2 and Stau1 in RNA granules in dendrites, but did affect the dynamics of RNG105 (Caprin1). In contrast to the belief model that FUS and TDP-43 harden RNA granules, the mobility of RNG105 was significantly increased, resulting in the release of RNG105 from RNA granules into the cytoplasm of dendrites. Simultaneously, the amount of mRNAs contained in RNA granules decreased, and local translation in/near the granules also decreased (Figure 2). Since RNG105 is responsible for mRNA recruitment to RNA granules and mRNA transport to dendrites, increased mobility and loss of localization of RNG105 in dendritic RNA granules may affect these functions, leading to impaired synapse formation and cognitive function.
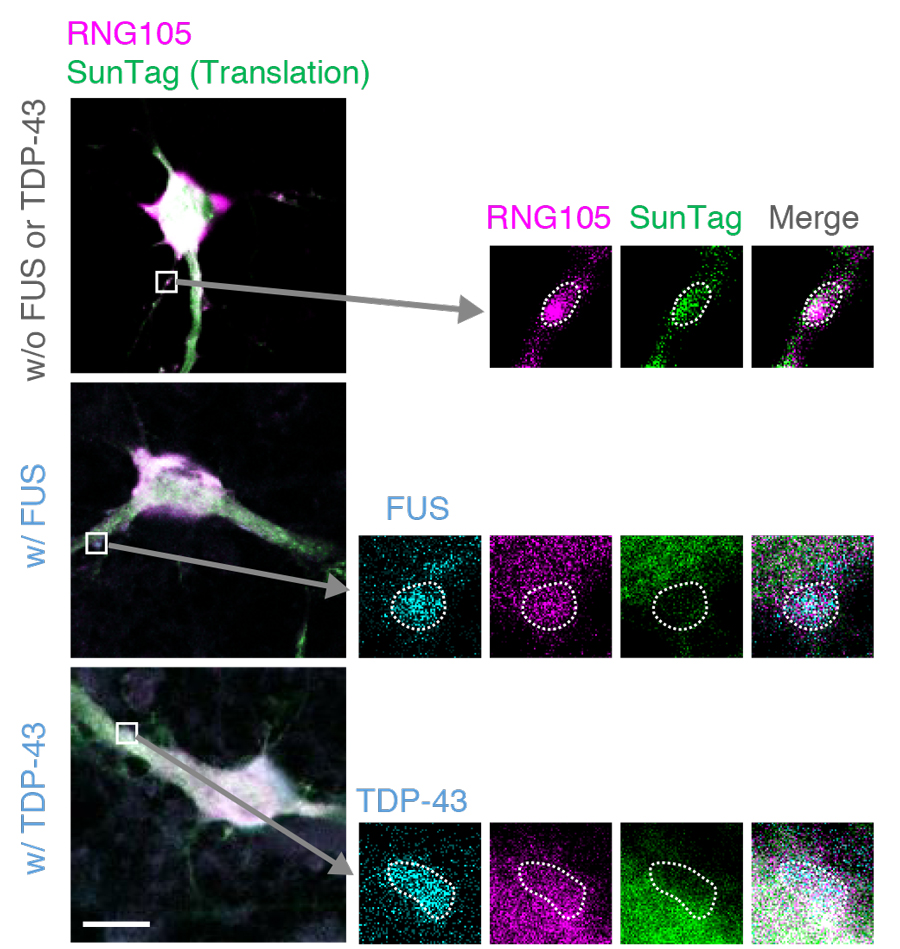
Figure 2. Accumulation of FUS and TDP-43 reduces translation in/near RNG105-localizing RNA granules in dendrites. Primary cultured neurons expressing RNG105-mRFP1 with and without co-expression of FUS-Sirius or TDP-43-Sirius were analyzed using the SunTag system to detect translation activity spatiotemporally. The boxed areas containing dendritic RNA granules are magnified in the right panels. The dotted lines outline the RNA granules. Scale bar, 10 μm.
III. Transport and local translation of mRNAs encoding the regulators of the small G protein Arf in neuronal dendrites
We have previously reported that RNG105 knockout in mice reduces mRNA transport to dendrites and impairs synaptic long-term potentiation and long-term memory (Nakayama et al., eLife, 2017). Gene ontology enrichment analysis of mRNA with reduced transport to dendrites in RNG105 knockout mice revealed that a group of mRNAs encoding regulators of the small G protein Arf (Arf GAPs and GEFs) was remarkably reduced in amount in the dendrites of RNG105 knockout neurons. Of these mRNAs, Psd mRNA, which encodes an Arf GEF, was most significantly reduced. In order to clarify the biological significance of dendritic transport of Psd mRNA, we are aiming to generate mice in which Psd mRNA transport can be artificially controlled.
In this study, we identified the 3'UTR of Psd mRNA as a cis-element responsible for the transport to dendrites. When Psd mRNA lacking the 3'UTR was expressed in mouse primary cultured neurons, the transport of that mRNA to dendrites was reduced. However, it was revealed that the 3'UTR was required not only for dendritic transport but also for translation. Therefore, we narrowed down the responsible regions for the transport and translation in the 3'UTR, and found that 5' half and 3' half of the 3'UTR were required for transport and translation, respectively. Based on these results, we are proceeding with the generation and analysis of mice in which only the 5' half of the 3'UTR of Psd mRNA is deleted to reduce only mRNA transport while maintaining the translation level of Psd mRNA.