Rhizobium–legume symbiosis is one of the most successful mutually beneficial interactions on Earth. In this type of symbiosis, soil bacteria called rhizobia supplies the host legumes with ammonia produced through bacterial nitrogen fixation. In return, host plants provide the rhizobia with their photosynthetic products. To accomplish this biotic interaction, leguminous plants develop nodules on their roots. However, more than 70% of land plant families have symbiotic relationships with arbuscular mycorrhizal (AM) fungi. Despite marked differences between the fungal and bacterial symbioses, common genes are required for both interactions. Using a model legume, Lotus japonicus, we are trying to elucidate the molecular mechanisms of both symbiotic systems.
Research Projects
-
• Root nodule
-
• Symbiosis
-
• Autoregulation of nodulation
-
• Long-distance signaling
-
• Lotus japonicus
-
• Arbuscular mycorrhizal fungi
I. Root nodule symbiosis
Legumes develop
de novo organs known as root nodules to accommodate symbiotic bacteria called rhizobia. Nodule formation involves two distinct processes: rhizobial infection in epidermis and nodule development accompanied by cell division in cortex (Figure 1).
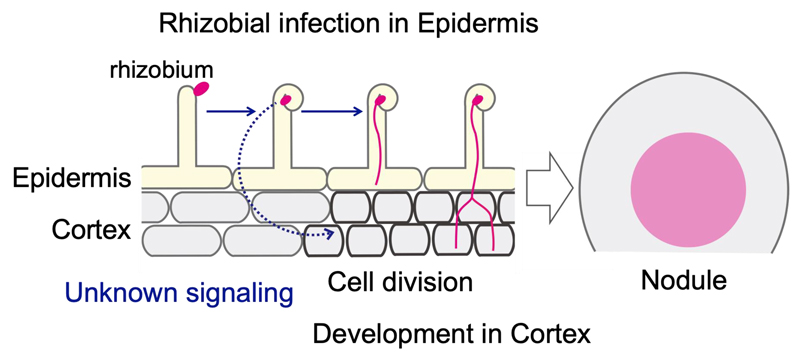
Figure 1. Processes for nodule formation.
The cortical cell division provides an indispensable scaffold for rhizobia progression from epidermis to cortex (Figure 1; pink line), leading to successful nodule formation. Cortical cell division occurs just below the site of rhizobial infection in epidermis. Therefore, there appears to be a spatiotemporal coordination across epidermis and cortex in this symbiotic organogenesis. Epidermal expression of genes required for early symbiotic genes is sufficient for nodule development in cortex, suggesting that some kinds of signals generated in epidermis trigger cortical cell division (Figure 1; blue dotted arrow). However, little is known about the mechanism that coordinates these two events.
In this study, we conducted a time-course transcriptome analysis using a
L. japonicus non-nodulation mutant “
daphne”, which has uncoupled symbiotic events in epidermis and cortex, in that it promotes excessive rhizobial infection in epidermis but does not produce nodule primordia in cortex. Among genes that showed different expression patterns in
daphne and wild type, we found
IAA CARBOXYL METHYLTRANSFERASE 1 (IAMT1), which encodes the enzyme that specifically converts auxin (indole-3-acetic acid; IAA) into its methyl ester (MeIAA). A significant MeIAA increase after rhizobial infection was detected by using
daphne roots, in which excess rhizobia are infected (Figure 2).
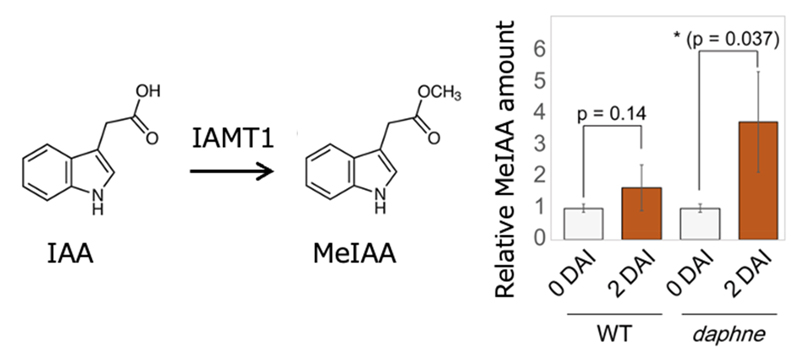
Figure 2. Auxin methylation in nodule symbiosis. IAMT1 converts IAA into MeIAA (left). Relative MeIAA amount of wild type (WT) and
daphne at 0 (non-inoculation) or 2 d after inoculation (DAI) (right).
In
Arabidopsis, IAMT1 reportedly serves development and differential growth of shoot. On the other hand, we found that
IAMT1 is duplicated in the legume lineage, and one of the duplicates (named
IAMT1a) was expressed by rhizobial infection in root of
L. japonicus.
LjIAMT1a-knockdown inhibited nodule formation (Figure 3).
LjIAMT1a overexpression promoted MeIAA accumulation and nodule formation. In contrast, overexpression of
LjMES17, a MeIAA esterase, decreased MeIAA levels and nodule number. These showed that auxin methylation is essential for nodule formation.
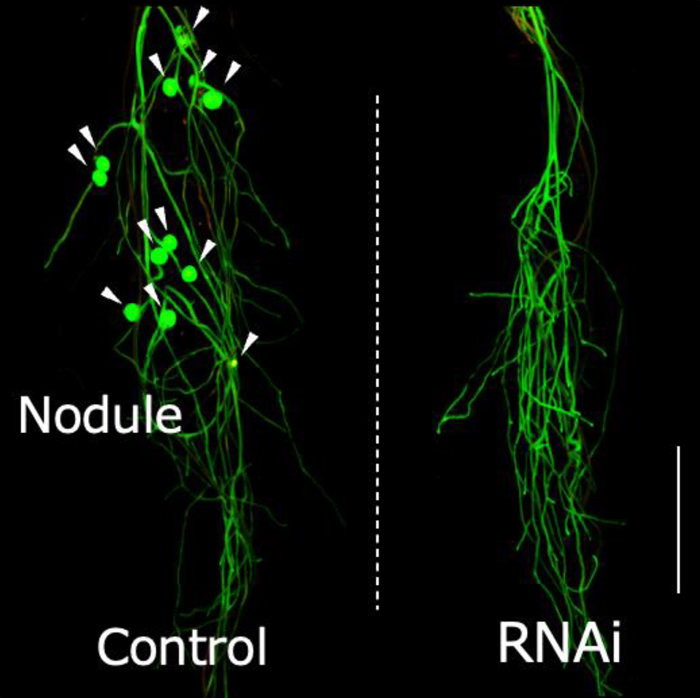
Figure 3.
LjIAMT1a-knockdown inhibited nodule formation. Hairy roots harboring empty vector (for control; left) or
LjIAMT1a-RNAi vector (for knock-down; right). Nodules are indicated by arrowheads. (Scale bars, 1 cm.)
Notably, application of MeIAA significantly induced expression of a symbiotic gene,
NIN, without rhizobia (Figure 4).
NIN is a key regulator for cortical cell division; therefore, auxin methylation could act on the process of cortical cell division. Whereas
IAMT1a was expressed mainly in root epidermis, it would be interesting to see how MeIAA affects cortex. The result also suggests that auxin methylation is not simply due to alteration of auxin homeostasis, contrary to what many studies assumed, MeIAA, as well as other auxin secondary metabolites, is also an inactive molecule of IAA. Understanding the function of auxin methylation and MeIAA in nodule development should open a new avenue for auxin metabolisms in the plant biology.
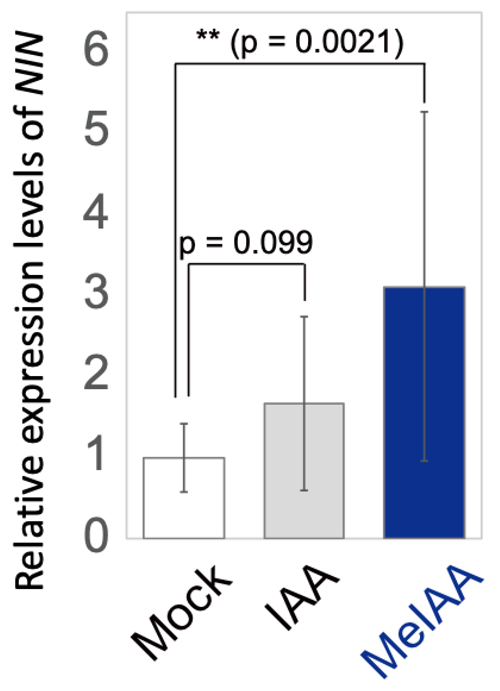
Figure 4. Exogenous MeIAA significantly induced
NIN expression without rhizobia. Relative expression levels of
NIN after treatment with DMSO as mock (white bar), IAA (gray bar), or MeIAA (blue bar) for 24 h.
II. Arbuscular mycorrhizal symbiosis
Arbuscular mycorrhizal (AM) symbiosis is the oldest beneficial mutualistic relationship between the majority of terrestrial plants and AM fungi. AM fungal spores germinate and elongate their hyphae toward the root of host plants then enter the root cells and form finely branched structures, arbuscules, for exchanging nutrients with plants (Figure 5). AM fungi deliver mineral nutrients especially phosphorus in the soil to host plants. In return, they obtain organic carbon sources such as sugars which are produced through photosynthesis. AM fungi are obligate symbionts depending on their host plants for essential nutrients, thus they are incapable of propagation by themselves.
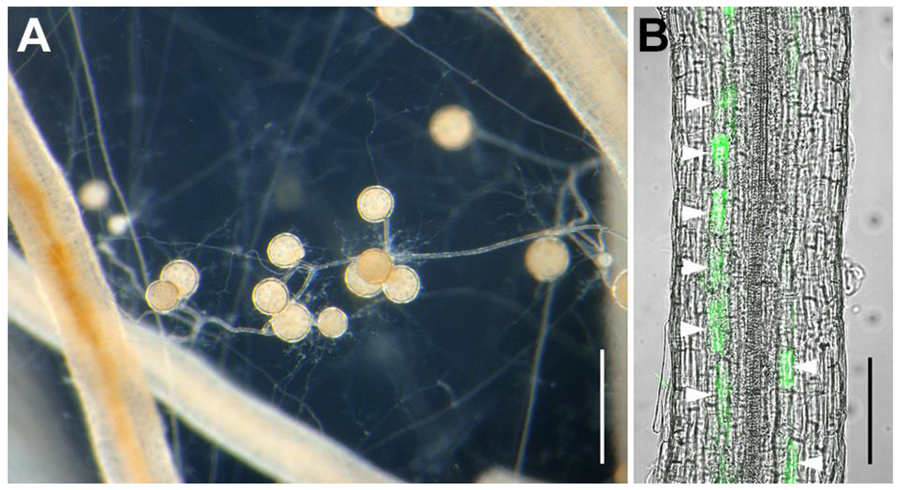
Figure 5. AM fungus
R. clarus HR1 growing with carrot hairy roots. (A) Expanded extraradical hyphae and formed symbiotically generated spores between the roots. Bar, 500 µm. (B) Fungal structures stained by green fluorescent (Wheat germ agglutinin Oregon green 488) in the root. Arrowheads, arbuscules inside root cortical cells. Bar, 100 µm.
In the history of AM fungal culture research, it was shown that an AM fungus
Rhizophagus irregularis could complete its life cycle in co-culture with mycorrhiza-helper bacterium
Paenibacillus validus in the absence of host plants. Over the last several years, it was reported that AM fungi receive not only sugars but also fatty acids. Subsequently, from these experiments, it was demonstrated that some fatty acids such as palmitoleate and myristate induce
R. irregularis spore formation under an asymbiotic (completely host-free) culture media.
We found that a fungus isolated from Aichi prefecture,
Rhizophagus clarus HR1, whose genome has been sequenced, shows better growth on the asymbiotic culture medium, thus is more suitable for this culture method. A fatty acid, myristate promotes
R. clarus hyphal growth, but the addition of only myristate could not ensure induction of asymbiotically-generated spores (AS) formation. Furthermore, the number of AS in this culture was much smaller than those of symbiotically-generated spores (SS) which are produced in the co-culture system with hairy roots.
Some phytohormones have been reported that they have important roles as signals during AM symbiosis. Especially, strigolactone is well known as a major signal which induces hyphal elongation and branching and stimulates their mitochondrial activity in the pre-symbiotic stage. By supplementing synthetic strigolactone, GR24 with myristic acid, the time of germination and AS formation in
R. clarus was accelerated. Although not all
R. clarus spores formed AS in the medium supplemented only with myristic acid, the addition of GR24 resulted in a quite high efficiency of AS formation to nearly 100 %. Besides strigolactone, it was reported that there was an increase of jasmonate levels in AM roots and the upregulation of genes involved in jasmonic acid biosynthesis in the cells containing arbuscules. Application of jasmonates in the medium with myristic acid and GR24 led
R. clarus to produce a higher number of AS with larger sizes (Figure 6).
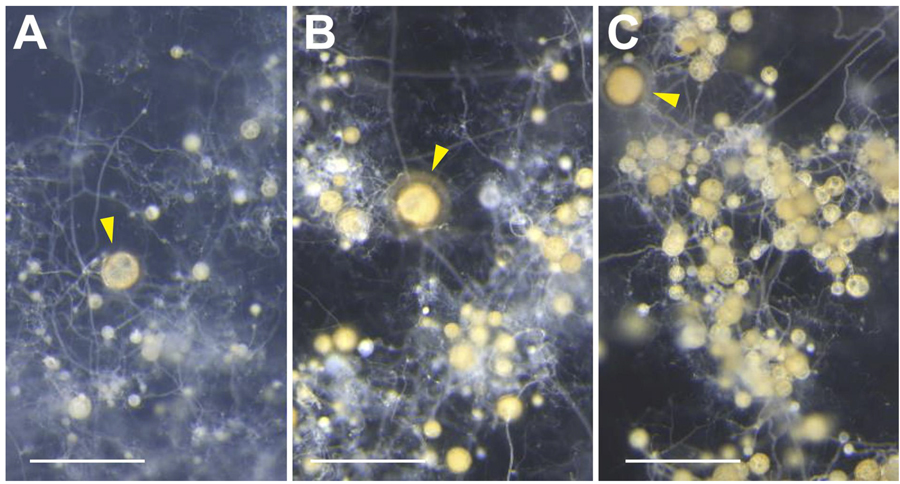
Figure 6. Produced spores on asymbiotic culture medium, supplemented with only myristate (A), myristate and GR24 (B), myristate, GR24 and methyl jasmonate (C). Arrowheads, parent spores. Bars, 500 µm.
Although the number of AS produced in the medium with myristate, GR24 and jasmonate has been close to that of SS, the size of AS is still smaller than SS. However, AS can be subcultured with the same efficiency as the first asymbiotic culture. Moreover, AS was capable of infecting hairy roots and plants. The growth of Welsh onions was significantly promoted by inoculation with AS as well as with SS (Figure 7). Our findings would lead us to more extensive studies of AM symbiosis and to the application of AS as an inoculum for basic research and for sustainable agriculture.
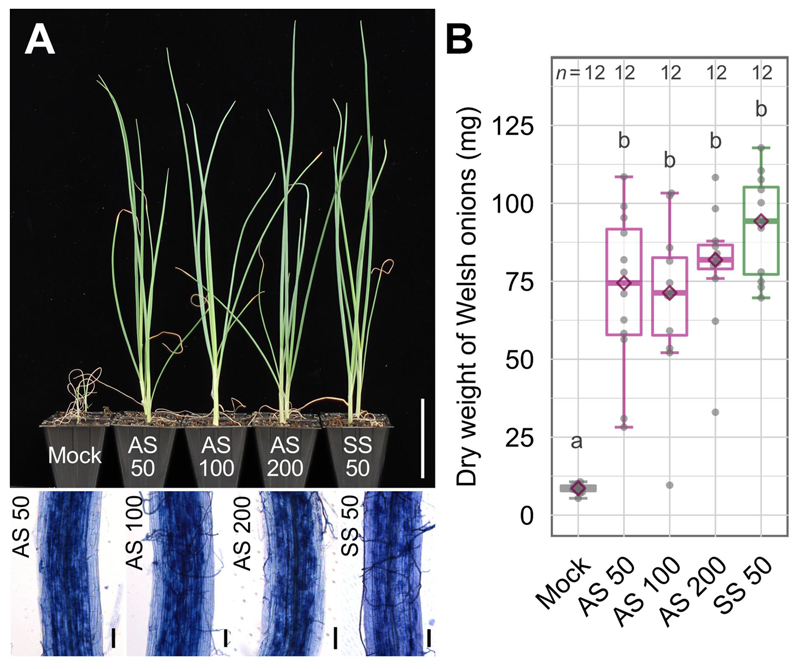
Figure 7. Inoculation of AM fungal spores to Welsh onions. Numbers following AS or SS are the number of spores for inoculation. (A) An appearance of plants inoculated with AM spores (upper). Bar, 5 cm. Ink-stained fungal structures in the roots (bottom). Bars, 50 µm. (B) Dry weight of Welsh onion shoots.
Different letters indicate significant differences among treatments in each trial using Wilcoxon rank-sum test with Bonferroni correction,
p < 0.05.