
the Venus flytrap Dionaea muscipula eating a cricket in the locality
ハエトリソウ属は、葉身が変化した捕虫器で小動物を捕らえて栄養とすることで、貧栄養地でも生育できる。上の写真は自生地で撮影したものだが、閉じた葉を開けるとコオロギが入っていた。同じ食虫植物であるモウセンゴケ属の姉妹属で、ハエトリソウDionaea muscipula1種からなる単型属である。
The Venus flytrap genus, Dionaea, captures small animals using modified leaves that act as a trap, obtaining nutrients to thrive in nutrient-poor environments. It is a sister genus to the sundew genus, which is also a carnivorous plant, and consists of a single species, Dionaea muscipula, making it a monotypic genus.
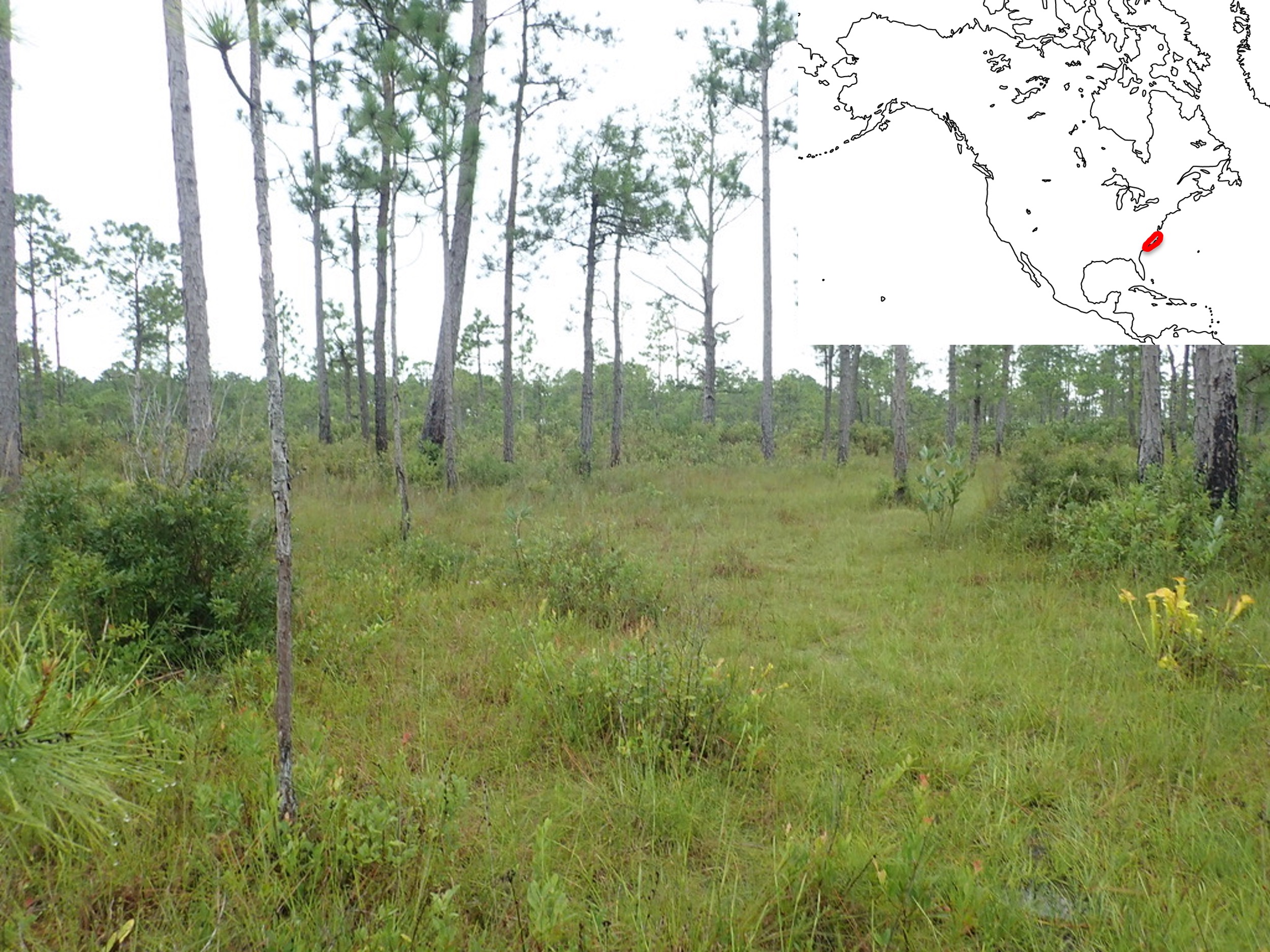
Locality of the Venus flytrap Dionaea muscipula near Wilmington, North Carolina, USA
ハエトリソウは米国のノースカロライナ州とサウスカロライナ州の海岸線の沿った約340 kmの範囲に分布していたが、土地開発や採集によって現在では限られた国立公園や私有地などで見られるだけである(Bailey and McPherson 2012, Juniper et al. 1989, Roberts and Oosting 1958)。地図は次のサイトより引用:https://www.freemap.jp/free.html
The Venus flytrap was distributed along a roughly 340 km stretch of the coastal region of North Carolina and South Carolina in the United States, but due to land development and poaching, it can now only be found in limited areas such as national parks and private lands (Bailey and McPherson 2012, Juniper et al. 1989, Roberts and Oosting 1958). The map was sited from https://www.freemap.jp/free.html
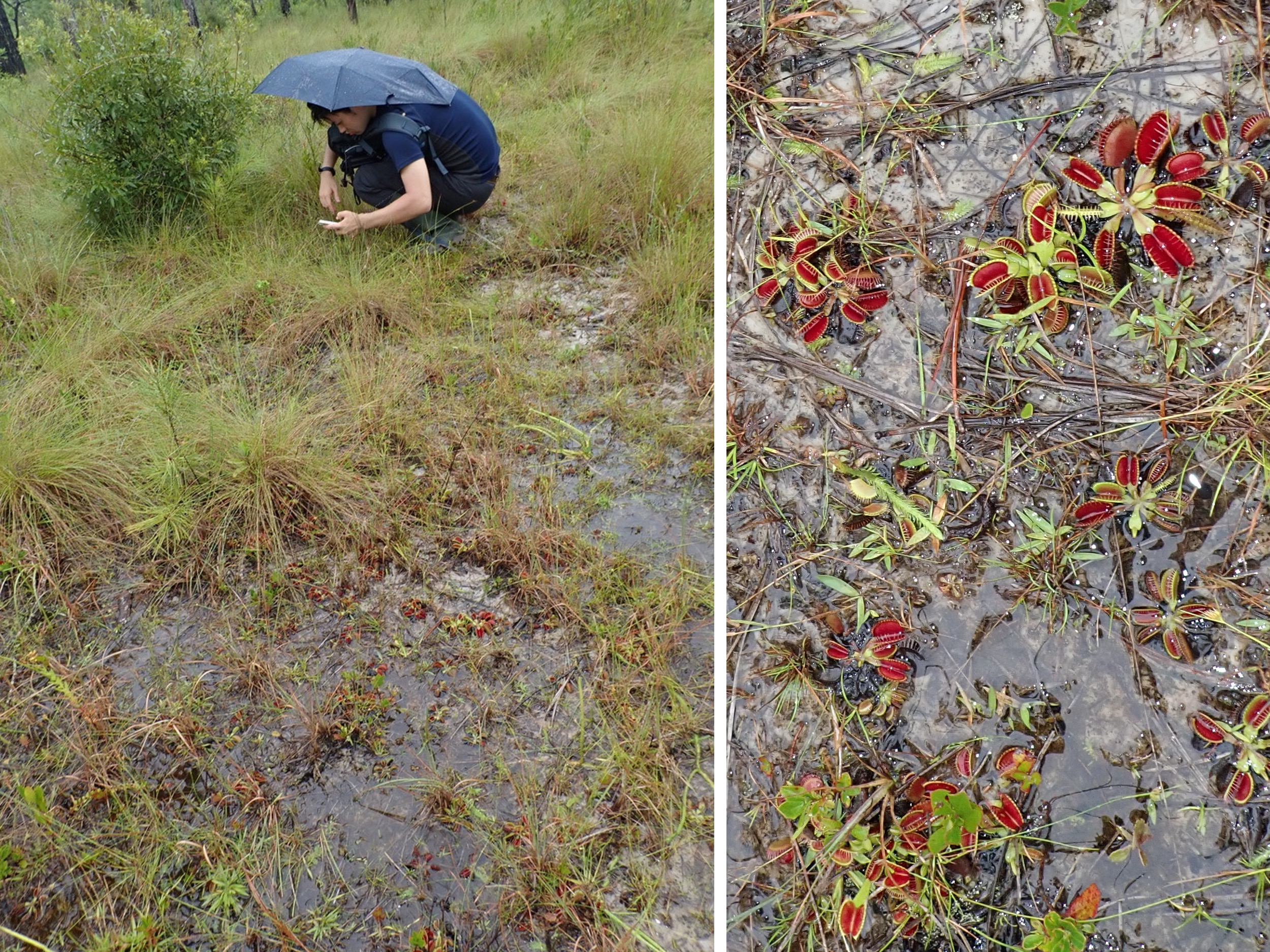
Locality of the Venus flytrap Dionaea muscipula near Wilmington, North Carolina, USA
ハエトリソウは、山火事が数年おきにおこる場所に生育する。地下の鱗茎は表面を焼く程度の山火事には耐性があり、上部を覆っていた草が焼き払われると元気に生育する。山火事の後には約10%開花個体が増えるが、数年して周りをイネ科、カヤツリグサ科、イグサ科の草本が覆うようになると開花数が減る(Bailey and McPherson 2012, Roberts and oosting 1958)。
The Venus flytrap grows in areas where wildfires occur every few years. The underground bulb is resistant to wildfires that burn only the surface, and it thrives once the grasses covering the top are burned away. After a wildfire, the number of flowering individuals increases by about 10%, but as grasses from the Poaceae, Cyperaceae, and Juncaceae families cover the area over the years, the number of flowering plants decreases (Bailey and McPherson 2012, Roberts and Oosting 1958).
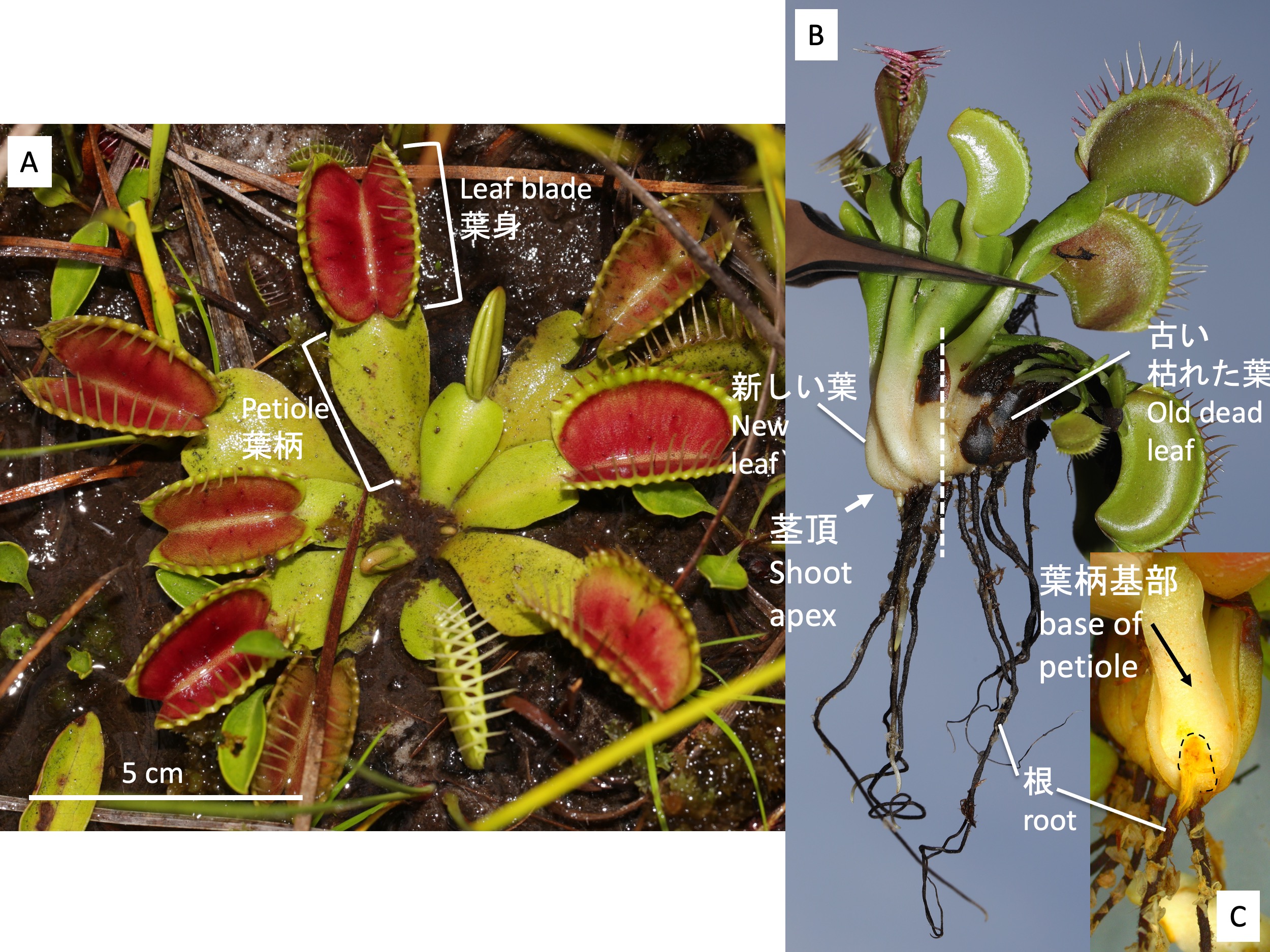
Morphology of the Venus flytrap Dionaea muscipula
ハエトリソウの葉は、葉身が捕虫器となり、葉柄が幅広に成長して普通の植物の葉身状になっている(A)。茎は地表近くを横走し、葉と葉の間(節間)が短いので、葉はロゼット状に展開する。葉柄基部は柔組織で肥厚し、地下部は鱗茎状になる。(C)は(B)の白色点線部分で切断した断面を茎の先端側(茎頂側)から写したものだが、細い茎(黒色点線で囲んだ部分)から葉柄と根が出ているのがわかる。
The leaves of the Venus flytrap have their leaf blades modified into traps, while the petioles grow broadly and take on the appearance of ordinary leaf blades (A). The stem runs horizontally near the surface of soil, and because the internodes between the leaves are short, the leaves form a rosette. The base of the petioles thickens with soft tissue, and the underground part becomes bulbous. (C) is an image of a cross-section of (B), cut along the white dotted line, taken from the shoot apex side of the stem. You can see that the petioles and roots emerge from the thin stem (enclosed in the black dotted line).
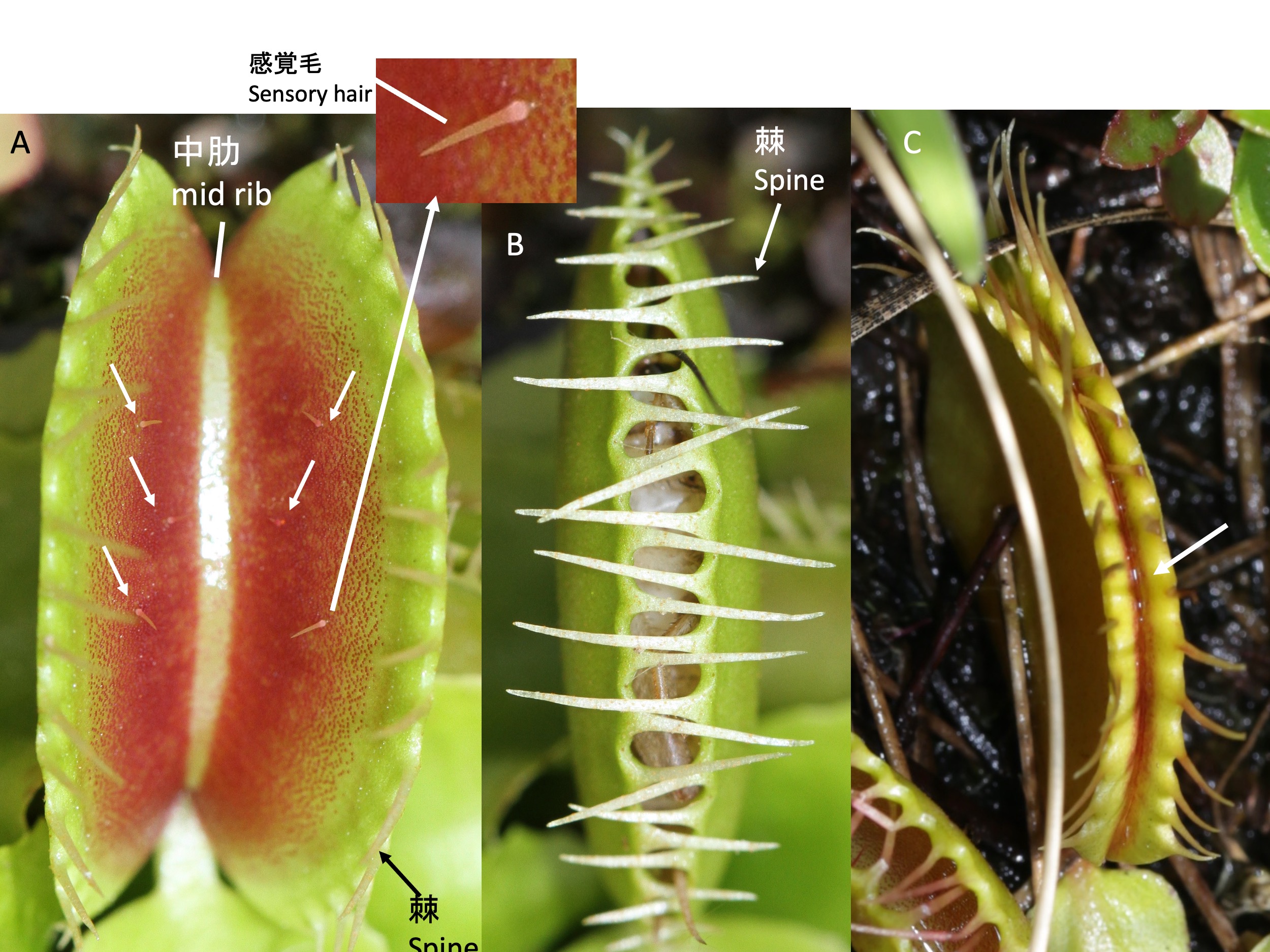
Trap morphology of the Venus flytrap Dionaea muscipula
捕虫器(A)の縁(葉身の葉縁)には棘(とげ)、表面には通常6本の感覚毛(Aの白色矢印)が形成される。感覚毛に、2回接触刺激が加わると、捕虫器が閉じ、獲物を捕らえる(B、ムービー)。葉が閉じた後、獲物がもがき、さらに感覚毛を刺激すると、葉身が背側に反り返って、獲物を圧迫するとともに、葉縁の少し中肋よりの部分が密着する。感覚毛への刺激は消化液の分泌を誘導し、密着した葉縁の内側は消化液で満たされる(Cの白矢印部分に消化液が漏れて光って見える)。
Spines form along the edges (the leaf margin) of the trap (A), and typically six sensory hairs (white arrows in [A]) develop on its surface. When the sensory hairs receive two successive touch stimuli, the trap closes, capturing the prey ([B] and Movie). After the trap closes, if the prey struggles and further stimulates the sensory hairs, the leaf blade bends backward, pressing the prey, and the area just inside the leaf margin seals tightly. The stimulation of the sensory hairs induces the secretion of digestive fluids, and the inner side of the tightly sealed leaf becomes filled with the digestive fluid (the white arrow in [C] indicates where the leaked digestive fluid is visible).
The trap of the Venus flytrap Dionaea muscipula close with two touch stimuli by Dr. Hiraku Suda
ハエトリソウを使った記憶に関する研究を紹介したい(長谷部2023より一部改変して引用)。植物に記憶とはなんぞやと思われるかもしれない。我々の記憶は脳によって司られる。では、脳の無い植物は記憶できないのだろうか。
Macfarlane(1902)は、ハエトリソウの感覚毛を1回だけ刺激しても葉が閉じないけれど、20秒間隔で2回刺激すると閉じることを発見した。このことは、ハエトリソウが1回目の刺激を記憶していることを意味している。さらに、1回目と2回目の刺激の間隔を30秒に延ばすと葉が閉じないことから、記憶は30秒ほどしかもたないことにも気づいた。そして、BrownとSharpは記憶時間が温度に依存することに気づいた(Brown and Sharp, 1910)。このことは、記憶が化学反応によっていることを示唆している。Brown (1916)はより定量的な実験を行い、10枚の葉に33秒間隔で2回の刺激を与えると、ほぼ全ての葉が閉じるが、67秒間隔だと平均2.75回の刺激が必要であると報告し、この実験に基づき、ハエトリソウは約30秒の記憶があると記載されることが多い。また、葉が2回の刺激で閉じる仕組みは、落ち葉、小石、雨などの獲物以外の物が偶然あたっても葉を閉じない点で適応的だと考えられている(Poppinga et al., 2018)。特に、雨は数日おきに降るので、雨粒にあたって葉が閉じないようにすることは、エネルギーの損失を防ぐ上で重要だと考えられる。栽培しているハエトリソウにジョウロで水をかけても葉が閉じない。よく見ると、1粒目の雨粒があたると、表面張力によって、水が感覚毛の周りを覆ってしまう。なので、2粒目以降の雨粒があたっても刺激が弱くなっているようである。
植物は、外界からのさまざまな刺激を記憶することが知られている(Taiz et al. 2022)。例えば、乾燥や高温などへの順応、病気にかかると病害抵抗性が強くなる植物免疫、冬を記憶して春に花が咲く春化などである。これらの数日以上の長期間の記憶に対し、ハエトリソウが持つ短期記憶は、植物には稀であることから興味を持たれ、多くの研究が行われてきた。
Here I introduce research on memory using the Venus flytrap (partially modified and cited from Hasebe 2023 [in Japanese]). You might wonder, “What does memory mean in plants?”. Our memory is governed by the brain. So, does this mean that plants, which lack a brain, cannot have memory? In 1902, Macfarlane discovered that while stimulating the sensory hairs of the Venus flytrap once does not cause the trap to close, two stimuli given 20 seconds apart will. This suggests that the Venus flytrap “remembers” the first stimulus. Furthermore, when the interval between the first and second stimulus is extended to 30 seconds, the trap does not close, indicating that this memory only lasts for about 30 seconds. Brown and Sharp later found that the duration of memory depends on temperature (Brown and Sharp, 1910), which implies that the memory is governed by chemical reactions. In 1916, Brown conducted more quantitative experiments, reporting that nearly all of 10 traps closed when stimulated twice at 33-second intervals, but at 67-second intervals, an average of 2.75 stimuli were needed. Based on this experiment, it is often stated that the Venus flytrap has a memory of around 30 seconds. Additionally, the mechanism by which the trap closes after two stimuli is considered adaptive because it prevents the trap from closing accidentally due to non-prey objects like leaves, pebbles, or rain (Poppinga et al., 2018). Rain, in particular, falls only every few days, so preventing the trap from closing in response to raindrops is important for conserving energy. If you water a cultivated Venus flytrap with a watering can, the trap does not close. On closer inspection, when the first raindrop hits, surface tension causes water to cover the area around the sensory hairs. Therefore, subsequent raindrops appear to provide a weaker stimulus.
It is known that plants can “memorize” various environmental stimuli (Taiz et al., 2022). Examples include acclimation to drought or high temperatures, plant immunity that strengthens after exposure to disease, and vernalization, where plants memorize winter and bloom in spring. While these examples are forms of long-term memory, lasting days or more, the short-term memory of the Venus flytrap is rare in plants and has thus attracted much research attention.
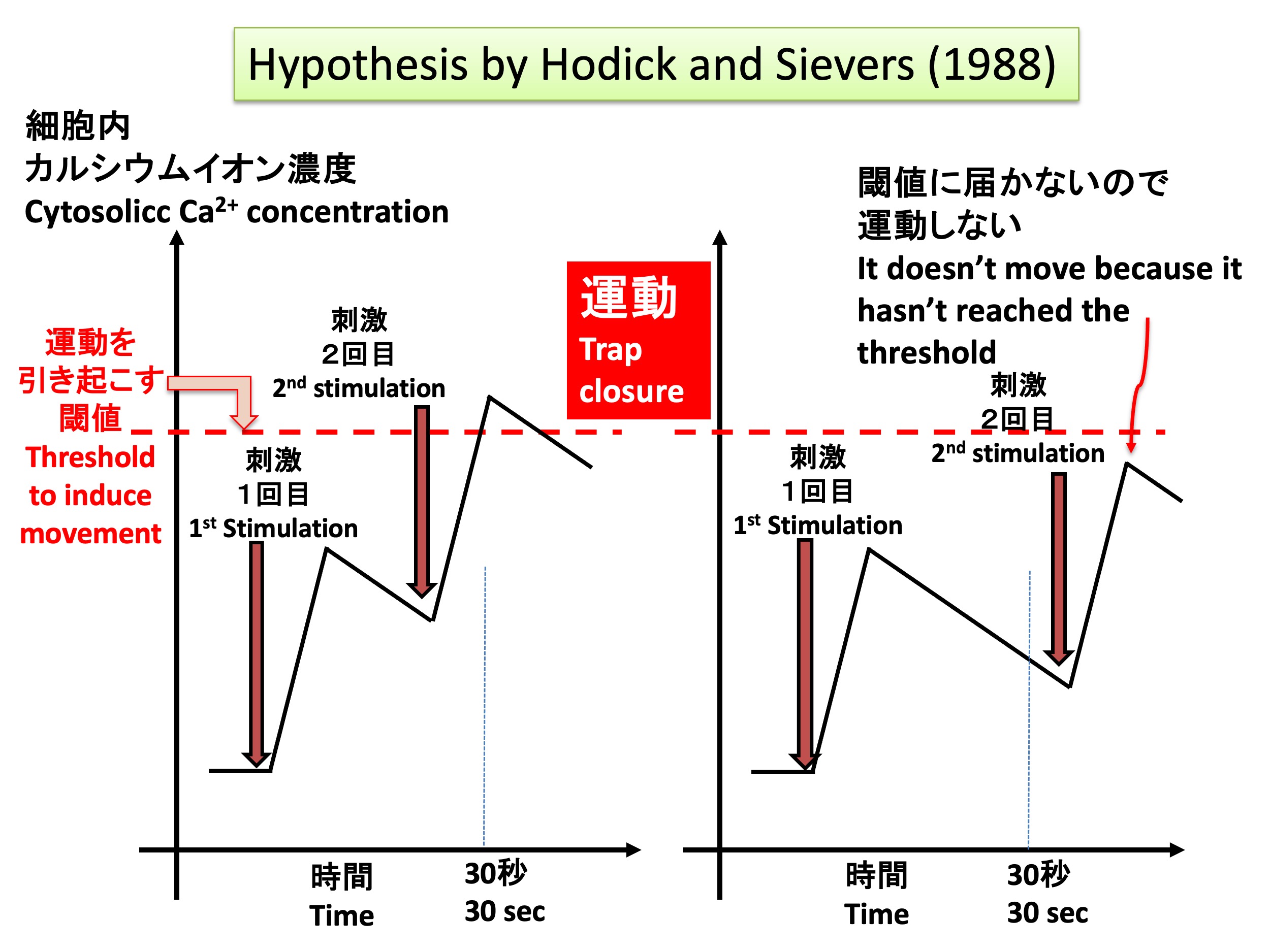
Hypothesis of trap movement of the Venus flytrap Dionaea muscipula by Hodick and Sievers (1988)
感覚毛を刺激すると、神経細胞のように、活動電位と呼ばれる一過性の電位変化がおこる(Burdon-Sanderson, 1873)。活動電位は葉が閉じる前に伝搬し(DiPalma et al., 1961)、感覚毛に刺激を与えると、感覚毛から葉全体に同心円状に活動電位が伝搬する(Sibaoka, 1966)。これらのことから、感覚毛から葉に広がる活動電位が刺激となり、なんらかの仕組みを駆動し、運動が引き起こされると考えられるようになった。
動物細胞では、活動電位が細胞膜にあるカルシウムチャネル(特定の条件によってカルシウムイオンを通すタンパク質)を制御することで、細胞質のカルシウムイオン濃度が変化する。カルシウムイオンは二次情報伝達物質として機能し、リン酸化などを介して他のタンパク質の機能を制御することで、細胞に変化を引き起こす。植物でも、巨大細胞を持つホシツリモを用いた研究から、活動電位によってカルシウムチャネルが活性化され、細胞外から細胞内へカルシウムイオンが流入する(Shiina and Tazawa, 1987)。このことから、植物細胞においても、動物細胞同様に、カルシウムが二次情報伝達物質として機能すると考えられるようになり、実際、ミルフラスコモとミナミシャジクモにおいて、活動電位によって細胞質のカルシウムイオン濃度が上昇し、原形質流動が停止することが示された(Hayama et al., 1979)。
HodickとSievers (1988)は、このような研究結果と、自らによるハエトリソウの活動電位の発生機構の研究から、カルシウムイオン濃度変化によって記憶現象が説明できるのではないかという仮説を提唱した(上図)。この仮説は、1)感覚毛を刺激すると活動電位が発生し、活動電位によって細胞内のカルシウムイオン濃度が上昇する、2)カルシウムイオンあるいはそれによって制御される分子が閾値(限界値)を超えた量蓄積すると葉が閉じる、3)1回の刺激ではカルシウムイオンの上昇は閾値を超えることができず、閾値を超えるには、少なくとも2回の刺激が必要である、4)カルシウムイオン濃度は1回目の刺激で上昇した後、徐々に減少し、30秒以上たつと、2回目の刺激を与えてもカルシウムイオン濃度が閾値を超えることができない、というものである。しかし、HodickとSieversの仮説を検証するには、細胞内のカルシウムイオン濃度がどのように変化するかを観察することが必要である。しかし、ハエトリソウにおいて細胞内カルシウムイオン濃度を検出する方法が無く、提唱から30年以上に亘って、仮説の検証ができずにいた。
When the sensory hairs are stimulated, a transient change in electrical potential called an action potential occurs, similar to what happens in nerve cells (Burdon-Sanderson, 1873). The action potential propagates before the trap closes (DiPalma et al., 1961). When the sensory hairs are stimulated, the action potential spreads concentrically from the hairs throughout the entire leaf (Sibaoka, 1966). From these findings, it became understood that the action potential spreading from the sensory hairs to the leaf serves as a stimulus that triggers some mechanism, causing the movement.
In animal cells, action potentials regulate calcium channels (proteins that allow calcium ions to pass under certain conditions) located in the cell (plasma) membrane, changing the concentration of calcium ions in the cytoplasm. Calcium ions function as secondary messengers, regulating the function of other proteins through processes like phosphorylation, thereby inducing changes in the cell. Similarly, in plants, studies using Nitella flexilis, a giant-celled green algae, have shown that action potentials activate calcium channels, allowing calcium ions to flow from outside the cell into the cell (Shiina and Tazawa, 1987). This led to the understanding that calcium functions as a secondary messenger in plant cells, just as it does in animal cells. Indeed, in Chara corallina and Nitellopsis obtusa, it was shown that action potentials increase cytoplasmic calcium ion concentrations, which in turn stop cytoplasmic streaming (Hayama et al., 1979).
Hodick and Sievers (1988) proposed a hypothesis that changes in calcium ion concentrations might explain the memory phenomenon, based on these studies and their own research into the mechanism behind action potentials in the Venus flytrap (see figure above). Their hypothesis includes the following points: 1) Stimulation of the sensory hairs generates an action potential, which raises the intracellular calcium ion concentration. 2) When calcium ions or molecules regulated by calcium accumulate beyond a threshold, the trap closes. 3) A single stimulus does not raise the calcium ion concentration above the threshold; at least two stimuli are required. 4) After the calcium ion concentration rises with the first stimulus, it gradually decreases, and after more than 30 seconds, a second stimulus cannot raise the calcium ion concentration above the threshold.
However, in order to test Hodick and Sievers’ hypothesis, it is necessary to observe how the intracellular calcium ion concentration changes. Unfortunately, no method for detecting calcium ion concentrations inside Venus flytrap cells existed, and for over 30 years after the hypothesis was proposed, it remained untested.
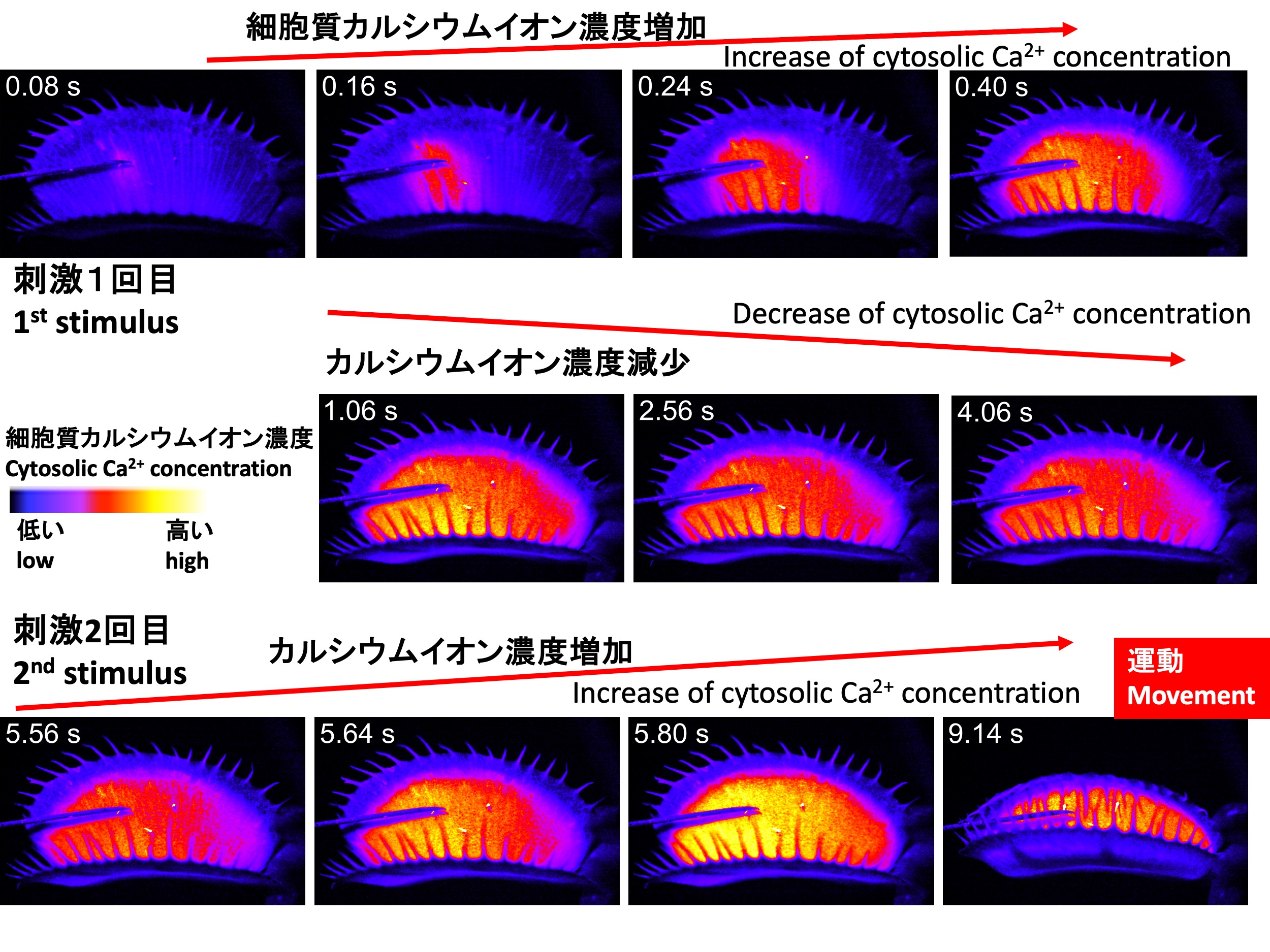
細胞内のカルシウムイオン濃度を検出するには、カルシウムイオンと結合すると光を発するようなセンサータンパク質をハエトリソウで働かせることが必要である。そのためには、センサータンパク質の遺伝子をハエトリソウに導入することが必要である。 そこで我々の研究グループの須田啓は、ハエトリソウにカルシウムイオンと結合すると緑色蛍光を出すGCaMP6fタンパク質をコードする遺伝子を導入し、遺伝子組換えハエトリソウを作出した。そして、感覚毛を1回刺激すると、感覚毛から葉全体に同心円状に緑色の蛍光が広がることを発見した(上図上段)。そして、広がった後、蛍光は時間がたつにつれ、徐々に減少した(上図中段)。2回目の刺激を与えると、1回目よりも強い蛍光が広がり、葉は閉じた(上図下段)(Suda et al., 2020)。
To detect intracellular calcium ion concentrations, it is necessary to use a sensor protein that emits light when it binds to calcium ions. This requires introducing the gene encoding the sensor protein into the Venus flytrap. Therefore, Dr. Hiraku Suda from our research group introduced a gene encoding the GCaMP6f protein, which emits green fluorescence when it binds to calcium ions, into the Venus flytrap, creating a genetically modified Venus flytrap, after mostly three years‘ efforts.
Upon stimulating the sensory hairs once, Suda discovered that fluorescence spreads concentrically from the sensory hairs across the entire leaf (imagens in the top row). After spreading, the fluorescence gradually decreased over time (images in the middle row). When a second stimulus was applied, a stronger fluorescence than after the first stimulus was observed, and the leaf closed (images in the bottom row) (Suda et al., 2020).
Cytosolic calcium ion dynamics of the Venus flytrap Dionaea muscipula with mechanical stimuli to the sensory hair (Taken by Dr. Hiraku Suda: Suda et al. 2020)
このムービーでは、最初の刺激を与えてから30秒以上たつと、二度目の刺激を与えても捕虫器は閉じないが、30秒以内だと閉じることを示している。最初の刺激から時間が経つにつれ、細胞内カルシウムイオン濃度に対応する黄色の蛍光がだんだん弱くなっていくのが観察できる。
1873年のBurdon-Sandersonの活動電位の測定に端を発したこれまでの研究の結果、HodickとSievers (1988)の仮説が正しく、ハエトリソウの記憶はカルシウムイオン量の変化で説明できる可能性が高い。しかし、今回の実験はあくまで、カルシウムイオン濃度変化と運動や記憶が「相互に関連している」ことを示してはいるが、カルシウムイオンが本当に機能しているかという「因果関係」はわからない。例えば、活動電位が我々の知らないカルシウムイオンとは全く別の物質を制御し、その物質がカルシウムイオンと同じ動態を示すかもしれない。因果関係を示すには、活動電位や他の物質を変化させずカルシウムイオン濃度だけを増減させることで運動や記憶がどう変化するかを生体内で観察することが必要であるが、活動電位発生にカルシウムイオンが関わっていること、活動電位やカルシウムイオンはさまざまなタンパク質を制御していることから、この実験は困難である。従って、今後、活動電位と細胞内カルシウムイオン変化がどのようなタンパク質や仕組みによって制御されているのか、細胞内カルシウムイオンがどのようなタンパク質をどのような化学変化によって変化させ、運動を引き起こすのかを明らかにすることが必要である。
In this video, it is shown that if more than 30 seconds pass after the first stimulus, the trap does not close upon the second stimulus, but if the second stimulus is given within 30 seconds, the trap closes. You can observe that the fluorescence, corresponding to the intracellular calcium ion concentration, gradually weakens over time after the first stimulus.
As a result of the research that began with Burdon-Sanderson’s measurement of action potentials in 1873, it is highly likely that the hypothesis of Hodick and Sievers (1988) is correct, and that the Venus flytrap’s memory can be explained by changes in calcium ion concentration. However, this experiment only shows that calcium ion concentration changes are “correlated” with movement and memory, not that calcium ions are actually functioning as the causal factor. For instance, it is possible that the action potential controls some unknown substance entirely different from calcium ions, and that this substance shows dynamics similar to calcium ions.
To demonstrate a causal relationship, it would be necessary to observe how movement and memory change in vivo by manipulating only the calcium ion concentration without altering the action potential or other substances. However, since calcium ions are involved in generating action potentials and both action potentials and calcium ions regulate various proteins, this experiment would be difficult. Therefore, future research needs to clarify how action potentials and intracellular calcium ion changes are regulated by proteins or other mechanisms, and how intracellular calcium ions induce movement by chemically modifying certain proteins.
Bailey T, McPherson S. 2012. Dionaea. Poole, Dorset, England: Redfern Natural History Productions Ltd.
Brown WH. 1916. The mechanism of movement and the duration of the effect of stimulation in the leaves of Dionaea. Am. J. Bot. 3: 68–90.
Brown WH, Sharp LW. 1910. The closing response in Dionaea. Bot. Gaz. 49: 290–302.
Burdon-Sanderson JS. 1873. Note on the electrical phenomena which accompany stimulation of the leaf of Dionaea muscipula. Phil. Trans. Roy Soc. London 164: 495.
DiPalma JR, Mohl R, Best Jr W. 1961. Action potential and contraction of Dionaea muscipula. Science 133: 878–879.
Hayama T, Shimmen T, Tazawa M. 1979. Participation of Ca2+ in cessation of cytoplasmic streaming induced by membrane excitation in Characeae internodal cells. Protoplasma 99: 305–321.
Hodick D, Sievers A. 1988. The action potential of Dionaea muscipula Ellis. Planta 174: 8–18.
Juniper BE, Robins RJ, Joel DM. 1989. The Carnivorous Plants. London, Uk: Academic Press.
Macfarlane JM. 1902. Contribution to the history of Dionaea muscipula Ellis. Contr. Bot. Lab. Univ. Pennsylvania 1: 7–44.
Poppinga S, Bauer U, Speck T, Volkov AG. 2018. Motile traps. In: Ellison AM, Adamec L, eds. Carnivorous Plants. Oxford: Oxford Univ Press, 180–193.
Roberts PR, Oosting HJ. 1958. Responses of Venus Fly Trap (Dionaea muscipula) to Factors Involved in Its Endemism. Ecological Monographs 28: 193–218.
Shiina T, Tazawa M. 1987. Demonstration and characterization of Ca2+ channel in tonoplast-free cells of Nitellopsis obtusa. J. Membr. Biol. 96: 263–276.
Sibaoka T. 1966. Action potentials in plant organs. Symp. Soc. Exp. Biol. 20: 49–73.
Suda H, Mano H, Toyota M, Fukushima K, Mimura T, Tsutsui I, Hedrich R, Tamada Y, Hasebe M. 2020. Calcium dynamics during trap closure visualized in transgenic Venus flytrap. Nature Plants 6: 1219–1224.
Taiz L, Miller IM, Murphy A, Zieger E. 2022. Plant Physiology and Development, Seventh Edition. Sinauer Associates Inc.
長谷部光泰(2023) 「食虫植物 多様性と進化」 裳華房