Plant organs have the ability to sense various vectorial stimuli such as light, humidity, touch and gravity as well as reorient their growth direction so as to be in a suitable position to survive and acclimate to their environment. Our aim is to understand the molecular mechanism of environmental responses as they pertain to gravity in the main.
1. Gravity sensing mechanism in gravitropism
Most of the environmental factors surrounding organisms change frequently (e.g. temperature, light). Gravity, on the other hand, is almost constant on Earth, and all living things have evolved under the gravitational force. Most organisms sense this unidirectional acceleration force and respond in some way. Plant organs sense gravity as a directional environmental cue that they use to control their growth orientation and their body architecture in order to promote their survival, for example via efficient photosynthesis, reproduction, water absorption, and nutrient uptake; such growth responses are termed gravitropism. Plants shoots grow upward (negative gravitropism), whereas roots grow downward (positive gravitropism). By using Arabidopsis thaliana, a model plant suitable for molecular genetic analyses, we are studying molecular mechanisms of gravitropism.
Gravitropism of inflorescence stems in wild-type Arabidopsis plants (at 3000x speed)
Gravitropism of inflorescence stems in sgr2 mutant plants (at 3000x speed)
Specialized cells in plant organs can sense the direction of gravity. In Arabidopsis thaliana, shoot endodermal cells and root columella cells are responsible for the gravity sensing. These gravity-sensing cells contain high-density starch-accumulating amyloplasts, which can change their position and relocate according to the direction of gravity when plant organs are inclined. The relocation of amyloplasts elicits biochemical signaling that promotes directional auxin transport to the direction of gravity.
Amyloplast sedimentation in the columella cells of an Arabidopsis main root
Our current questions are;
1) How is amyloplast relocation converted into biochemical signals?
2) How does the signal regulate auxin transport?
3) How does gravitropism affect plant architecture?
1) How is amyloplast relocation converted into biochemical signals?
(Drs. Nishimura & Shikata)
To identify genes involved in the gravity signaling, we carried out transcriptome analysis focusing on the gravity-sensing cells and found that several LAZY1-LIKE (LZY) genes are specifically expressed in the gravity-sensing cells. LAZY1 was originally identified in rice (Oryza sativa) as the gene responsible for a mutation that causes shoot gravitropic defect. LZY family genes are conserved in most land plants, while they encode plant-specific proteins of unknown function. We have shown that LZY genes are required for gravity signaling in gravity-sensing cells following amyloplast relocation. LZY3 protein polarly localized to the bottom side of the plasma membrane of root columella cells. Interestingly, when the root was inclined, LZY3 was rapidly relocalized to the new bottom side of the cell. We are currently investigating the mechanisms underlying the dynamic behavior of LZY3 upon gravi-stimulation through molecular genetics, biochemical analyses, and live cell imaging using a vertical-stage confocal microscope.
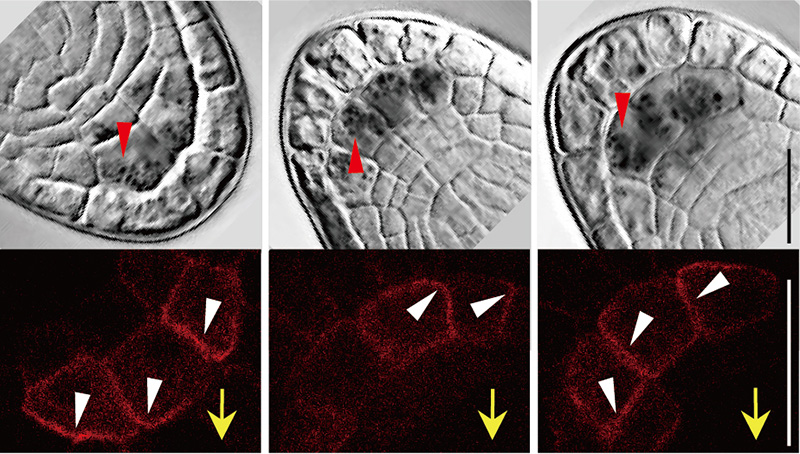
Subcellular localization of mCherry-fused LZY3 protein in the columella cells of lateral roots. Bofore rotation (left panel), 5 min after 180 degree-rotation (middle panel), and 60 min after the rotation (right panel).
2) How does the signal regulate auxin transport?
(Dr. Nishimura)
We found RLD/PRAF proteins as interactors of LZY proteins and demonstrated that RLD1 and RLD4 are also involved in gravity signaling process in root gravitropism. rld1;2;3;4 quadruple mutant displayed severe embryonic development defects due to a reduced amount and abnormal localization of PIN auxin efflux carriers, indicating that RLD family genes redundantly function in not only gravitropism but also plant development through regulation of auxin transport. Our data suggest that LZY3 recruit RLD proteins to the plasma membrane where RLDs regulate PIN proteins in gravity-sensing cells. We are currently investigating the molecular functions of RLD proteins in regulation of auxin transport.
3) How does gravitropism affect plant architecture?
(Dr. Kawamoto)
The overall plant architecture is determined by the growth angles of lateral organs, such as lateral roots and lateral branches. The branch growth angle affected by gravity is known as the gravitropic setpoint angle (GSA), and it has been proposed that the GSA is determined by the balance of two antagonistic growth components: gravitropism and anti-gravitropic offset (AGO). Among six LZY family genes in Arabidopsis, LZY1 is a major contributor in shoot gravitropism. It has been reported that TILLER ANGLE CONTROL 1 (TAC1) takes a part of AGO in shoot. TAC1 is a protein similar to LZY family, but lacks a C-terminal CCL domain which is essential for the interaction with RLD family proteins. We are investigating how LZY1 and TAC1 control gravitropism and AGO, and how antagonistic these growth components (gravitropism and AGO) led by LZY1 and TAC1 are integrated and balanced to coordinate growth angle control.
Besides LZY1 function in gravitropism, we are currently investigating the novel function of LZY1 in plant development. Recently we found that the LZY1 contributes not only gravitropism but also plant reproduction processes. Combining molecular genetics, biochemistry, cell biology, genomics, and also synthetic biology, we are approaching to elucidate the molecular mechanisms underlying the plant development.
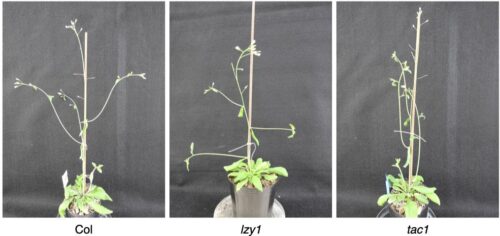
Plant architecture of wild-type (left), lzy1 mutant (middle) and tac1 mutant (right).
2. Re-orientation mechanism of the polarized growing root hair cell
(Dr. Shikata)
Root hairs, thin tubular cells grown outward from the root surface, increase root surface area and the uptake of nutrients and water from soils. They are typical polarized growing cells and grow straight and long in a cell-autonomous manner when they are grown in open air or within a liquid medium. However, soils are filled with soil particles like grains of sand of various sizes or silt, which may interfere with the growth of root hairs. We have developed an imaging system based on microfluidics to observe how root hairs grow in soils, since soils are not transparent enough to conduct observations within them through the use of microscopes. Applying this system, we have succeeded to visualize Arabidopsis roots including root hairs that come into contact with obstacles in the microfluidic device. We also have been analyzing various regulators that are important for normal root hair growth with the imaging system to understand the mechanism.
3. Optogenetic approaches in plant biology
(Dr. Shikata)
Optogenetics is a powerful tool to understand how proteins function in cells. Many optogenetic tools have been developed, however there are few applications in plant biology because of technical limitations. We have been trying to develop optogenetic tools which are applicable to cell biology in plants.